Energy Code Analysis: Modeling the Energy Performance of an Office Building
- Blanco Electric LTD. CO.
- Sep 1, 2023
- 8 min read
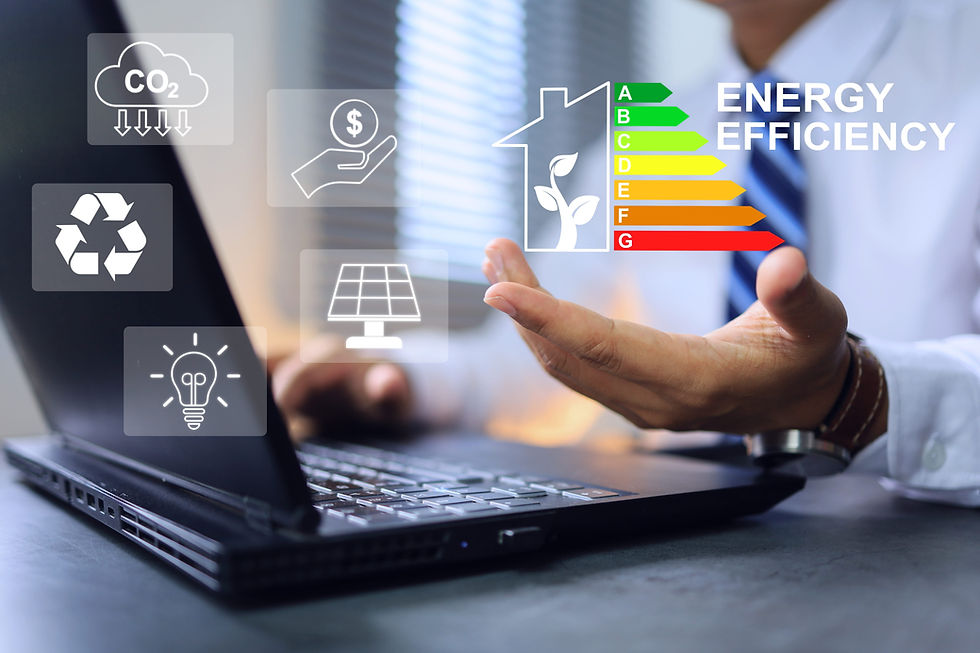
As a Houston electrical contractor, Blanco Electric offers Energy Code Analysis as part of its portfolio of electrical engineering services. Part of the ECA process applied to a building, we model the energy performance of this building. This is a systematic process that enables us to evaluate the energy consumption of all systems within the building.
This document outlines the detailed steps we follow to model the energy performance of a three-story office building located in Houston, TX, with the aim of assessing its energy efficiency and exploring potential retrofit scenarios.
1. Data collection
Step 1 is to gather all essential information and parameters that will form the foundation of the energy performance model. Without accurate and comprehensive data, any subsequent analysis would be based on assumptions, leading to potential inaccuracies in the model's predictions and recommendations.
Data collection begins with obtaining detailed architectural drawings of the building. These drawings provide insights into the building's geometry, layout, and structural components, which are crucial for understanding heat transfer, daylight penetration, and other energy-related factors. Systems specifications, on the other hand, offer a clear picture of the building's energy consumption patterns. By understanding the specifications and performance characteristics of HVAC, lighting, and other systems, the model can accurately simulate how these systems consume energy under various conditions.
Additionally, collecting occupancy and operational data is vital. Energy consumption in an office building is not just a function of its physical attributes but also how it's used. Knowing when and how spaces within the building are occupied, and how equipment is used, allows for a more precise modeling of energy loads.
Expected outcomes:
A comprehensive dataset that captures the building's physical characteristics and operational patterns.
Identification of potential data gaps or areas where assumptions might be needed.
A solid foundation upon which the subsequent steps of the energy modeling process can be built, ensuring that the model's predictions are as accurate and relevant as possible. It is also essential to validate and cross-check the information so gathered. This ensures that any inconsistencies or anomalies in the data are identified early on, preventing potential errors in the later stages of modeling.
Another crucial outcome is the establishment of a baseline. By understanding the current state of the building – its materials, systems, and usage patterns – we can measure any improvements or changes against this baseline. This is particularly valuable when assessing the impact of retrofit scenarios or energy-saving measures.
The data collected also serves as a reference point for stakeholders. It offers transparency, allowing everyone involved to understand the building's current state and the basis for subsequent analyses. This fosters trust in the modeling process and ensures that recommendations made later are grounded in reality.
Steps:
1.1 Architectural drawings: Obtain detailed architectural drawings of the building, including floor plans, elevations, sections, and site plans.
1.2 Systems specifications: Gather specifications for all energy-consuming systems, including HVAC, lighting, electrical systems, and appliances.
1.3 Occupancy and operational data: Understand the typical occupancy patterns, operational hours, and equipment usage schedules.
2. Preliminary analysis
Step 2 serves as the bridge between raw data collection and the detailed modeling process. It involves a high-level assessment of the collected data to identify key factors that will significantly influence the building's energy performance. This step ensures that the modeling process is tailored to the building's unique characteristics and challenges, allowing for a more accurate and relevant analysis.
Expected outcomes:
Informed modeling strategy: With insights from the preliminary analysis, the modeling process can be tailored to address specific challenges or opportunities identified for the building.
Baseline for comparison: The analysis provides a reference point against which the building's actual energy performance can be compared, helping identify areas of inefficiency or potential improvement.
Identification of key parameters: The preliminary analysis highlights critical factors that will significantly influence the building's energy consumption, ensuring they are given due consideration in the modeling process.
Stakeholder alignment: By presenting a high-level overview of the building's energy dynamics, stakeholders can align their expectations and understand the factors that will be crucial in the subsequent modeling steps.
In essence, Step 2 lays the groundwork for a focused and relevant energy modeling process, ensuring that the model is not just technically accurate but also contextually relevant to the building's specific environment and usage patterns.
Steps:
2.1 Building orientation: Analyze the building's orientation to understand its exposure to the sun.
2.2 Thermal zones: Identify and delineate thermal zones based on occupancy, usage, and exposure.
2.3 Climate data: Source local climate data for Houston, TX, to understand seasonal variations and typical weather patterns.
3. Modeling software selection
Step 3 is pivotal in determining the tools and platforms that will be employed to create, simulate, and analyze the energy performance model of the building. The choice of software can significantly influence the depth, accuracy, and granularity of the analysis.
Given the myriad of energy modeling software available, it's essential to select one that aligns with the building's requirements, the project's goals, and the available data.
Tailored analysis: Different software options cater to varying levels of complexity. While some are designed for quick, high-level assessments, others allow for in-depth, granular simulations. The choice should reflect the depth of analysis required for the project.
Integration capabilities: Some software platforms integrate seamlessly with architectural design tools or other simulation software, enabling a more streamlined workflow.
User interface and experience: The ease of use, visualization capabilities, and user experience can influence the efficiency and effectiveness of the modeling process.
Expected outcomes:
Optimized modeling process: By selecting the most appropriate software, the modeling process becomes more efficient, ensuring that the analysis is both thorough and timely.
Accurate and relevant simulations: The right software can simulate the building's energy performance with a high degree of accuracy, ensuring that the results are reliable and actionable.
Flexibility in analysis: Some software options offer modular capabilities, allowing for specific components or systems to be analyzed in isolation or in conjunction with others. This flexibility can be invaluable when exploring various retrofit scenarios.
Stakeholder confidence: By choosing a reputable and recognized software platform, stakeholders can have greater confidence in the modeling process and its results. They can be assured that industry best practices are being followed and that the analysis is grounded in proven methodologies.
Clear visualization and reporting: Modern energy modeling software often comes with advanced visualization tools, enabling stakeholders to understand the building's energy dynamics visually. This can be instrumental in communicating complex data in an accessible manner.
While there are multiple energy modeling software options available, we select the most appropriate one based on the building's requirements. Key differences among popular software include:
EnergyPlus: Highly detailed and customizable. Suitable for complex simulations.
eQUEST: User-friendly with a graphical interface. Ideal for quick simulations and preliminary assessments.
OpenStudio: Integrates with EnergyPlus and SketchUp, allowing for detailed modeling and visualization.
4. Building the model
Step 4 is the core phase where all the collected data and insights from the preliminary analysis are integrated into a digital representation of the building. This digital model serves as a virtual laboratory, allowing for simulations and assessments of the building's energy performance under various conditions.
Expected outcomes:
Comprehensive digital representation: At the end of this step, there exists a detailed digital twin of the building, capturing all its energy-related attributes. This model serves as the foundation for all subsequent simulations and analyses.
Baseline energy performance: With the model in place, an initial simulation can provide insights into the building's current energy performance, serving as a benchmark against which future improvements can be measured.
Identification of potential issues: As the model is built, any inconsistencies, data gaps, or potential issues can be identified. This early detection ensures that the model is both accurate and reliable.
Scenario preparedness: With a comprehensive model, various scenarios, such as changes in occupancy, equipment upgrades, or HVAC modifications, can be simulated. This flexibility is crucial for exploring retrofit opportunities and assessing their impact.
Stakeholder engagement: The visual nature of the model can serve as a powerful tool for engaging stakeholders. It allows for a clearer understanding of the building's energy dynamics and the potential areas of intervention.
Steps:
4.1 Geometry and envelope: At this stage, the physical structure of the building, including its walls, roofs, windows, and doors, is defined in the model. This helps in understanding how the building interacts with its external environment, particularly in terms of heat transfer and daylight penetration.
4.2 Internal loads: The energy demands within the building, such as those from occupants, lighting, and equipment, are specified. These internal loads play a significant role in determining the building's overall energy consumption.
4.3 HVAC systems: The heating, ventilation, and air conditioning (HVAC) systems are integrated into the model, defining how the building maintains its internal conditions in response to external and internal loads.
4.4 Lighting and electrical systems: These systems are crucial in determining the electrical energy consumption of the building. By modeling them accurately, we can assess opportunities for energy savings and efficiency improvements.
5. Simulation and analysis
Step 5 is the phase where the meticulously constructed digital model is put to the test. Through a series of simulations, we observe the response of the model to various conditions and scenarios. This step aims to understand the building's energy behavior, identify inefficiencies, and evaluate potential interventions.
Expected outcomes:
Detailed energy profile: Post-simulation, a comprehensive energy profile of the building is generated. This profile breaks down energy consumption by system, zone, and time, offering granular insights into the building's energy dynamics.
Identification of inefficiencies: The simulations can highlight areas where energy is being wasted or where systems are not operating optimally. These inefficiencies become targets for potential interventions.
Evaluation of retrofit scenarios: For each retrofit scenario simulated, a clear understanding of its impact on energy consumption, comfort levels, and cost savings is obtained. This information is crucial for decision-making, allowing stakeholders to prioritize interventions based on their goals and budget.
Predictive insights: Beyond understanding the current state, the simulations can offer predictive insights. For instance, how might the building's energy performance be affected by future changes in climate, utility rates, or occupancy patterns?
Data-driven recommendations: Based on the simulations and analyses, specific, data-driven recommendations can be formulated. These recommendations are grounded in empirical evidence, ensuring that they are both actionable and effective.
Steps:
5.1 Baseline simulation: Run the initial simulation to get the building's current energy performance.
5.2 Sensitivity analysis: Identify key parameters that significantly impact energy consumption.
5.3 Retrofit scenarios: Based on theoretical observations, simulate various retrofit scenarios such as upgrading insulation, replacing HVAC systems, or transitioning to LED lighting.
6. Results and recommendations
Step 6 is the culmination of the modeling and simulation processes. After rigorous simulations in the previous step, this phase is dedicated to interpreting the results, drawing meaningful conclusions, and formulating actionable recommendations. Our primary aim is to provide stakeholders with clear, data-driven insights and guidance on enhancing the building's energy efficiency.
Expected outcomes:
Clear Understanding of Energy Dynamics: Stakeholders will have a comprehensive understanding of the building's energy consumption patterns, allowing them to make informed decisions.
Benchmarking: With key efficiency metrics in hand, the building's performance can be compared to industry standards or peer buildings. This benchmarking can highlight areas of excellence or potential improvement.
Actionable Roadmap: The recommendations provide a clear roadmap for enhancing energy efficiency. Whether it's upgrading a lighting system, modifying operational schedules, or retrofitting insulation, stakeholders will know the expected impact and benefits of each intervention.
Return on Investment (ROI) Analysis: For each recommended action, an ROI analysis can be provided, allowing stakeholders to prioritize interventions based on their budget and desired payback period.
Enhanced Stakeholder Confidence: With data-driven insights and recommendations, stakeholders can have greater confidence in their decisions. They can be assured that proposed interventions are grounded in rigorous analysis and are tailored to their building's specific needs.
Steps:
6.1 Energy consumption breakdown: Provide a detailed breakdown of energy consumption by system and by zone.
6.2 Efficiency metrics: Calculate and present key metrics such as Energy Use Intensity (EUI).
6.3 Retrofit recommendations: Based on the retrofit simulations, recommend the most cost-effective and energy-efficient solutions.
7. Reporting
7.1 Executive summary: Provide a high-level overview of the findings, suitable for decision-makers.
7.2 Detailed analysis: Offer a granular analysis of the simulations, including charts, graphs, and tables.
7.3 Recommendations: List actionable steps for improving the building's energy efficiency.
Important information: This process is proprietary to Blanco Electric. It is communicated for informational purposes only and not intended to be copied or imitated. Do not copy or republish this document.
Comments